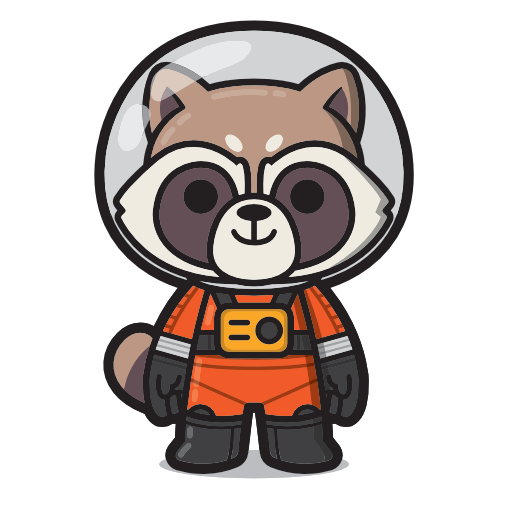
Spaceraccoon's Blog
InfoSec and White Hat Hacking
It’s always interesting to find edge cases in strong appsec programmes like Meta and Google that have generally solved entire bug classes like cross-site scripting because it highlights potential blind spots in appsec strategy. In particular, I’m still fascinated by the Clipboard API that seems to evade typical static analysis tools, like a stored XSS I found in Zoom Whiteboard. Here’s how I found similar bugs in Excalidraw (used in Messenger and other Meta assets) and Microsoft Whiteboard.
Have you ever wondered how display monitor software can change various settings like brightness over a simple display cable? As it turns out, this relies on a standard protocol that can lead to interesting vulnerabilities. Here’s how I found and exploited CVE-2023-5449 in dozens of HP display monitors.
The Offensive Security Exploitation Expert (OSEE) certification is a legendary apex achievement among OffSec’s offerings - unabashedly featuring a skull logo and grim reaper iconography in previous iterations. Here’s how I tackled it while busy at work.
One common perception is that it is easier to write rules for Semgrep than CodeQL. Having worked extensively with both of these static code analysis tools for about a year, I have some thoughts.
When is copy-paste payloads not self-XSS? When it’s stored XSS. Recently, I reviewed a Zoom’s code to uncover an interesting attack vector.